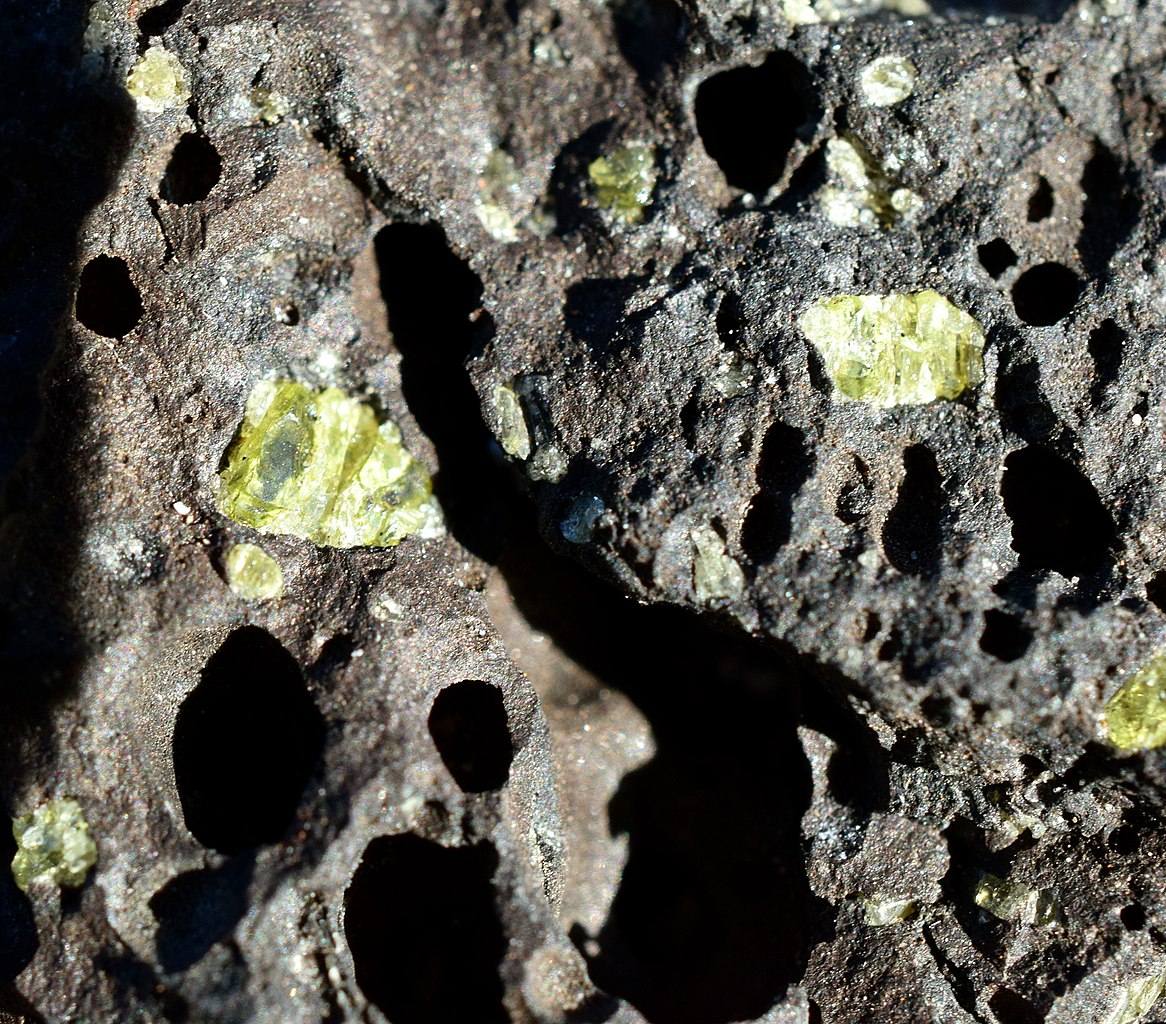
KEY CONCEPTS
- Rocks are solid masses of naturally occurring geological and related materials of many sorts.
- Petrology is the branch of geology concerned with the compositions, structures, and origins of rocks.
- Petrologic research involves both field studies and laboratory studies. It is a hybrid science that involves aspects of chemistry, mineralogy, physics, geological mapping, and sometimes biology.
- Petrography, a subdiscipline of petrology, deals specifically with the description and classification of rocks.
- Petrologic research involves examination of rocks in outcrops and hand samples, examining rocks using a petrographic microscope, and sometimes geochemistry.
- We commonly divide rocks into three classes: igneous rocks, sedimentary rocks, and metamorphic rocks.
- Rocks and minerals may change between being igneous, sedimentary, or metamorphic.
- Petrology is important because it tells us about Earth history, it is the key to discovery and development of mineral resources, and because fundamental principles and lessons learned from petrology have applications in modern industry.
1.1 Rocks
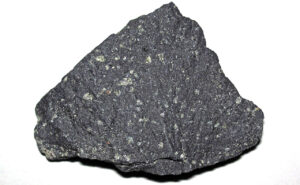
Figure 1.1 shows a glass-containing olivine basalt. The green olivine crystals are quite easily seen, but the glass is not. The opening photo in this chapter is a close-up picture of a different olivine basalt – one that contains lots of vesicles.
Although we all know a rock when we see one, rocks vary greatly. Typically, we think of rocks as mineral aggregates, but this is not always the case. Rocks may be partly or entirely made of fossils or other biological materials. They may be partly or entirely volcanic glass that, because it is not crystalline, is not a mineral. Rocks may also include pieces of other preexisting rocks. Perhaps the simplest definition is to say that a rock is a solid mass of naturally occurring geological and related materials of any sort.
Chapter 16 of this book contains a photo gallery with many photos of igneous and metamorphic rocks. If you want to see what they look like, go there. |
1.1.1 Petrology
Petrology is the branch of geology concerned with the compositions, structures, and origins of rocks. Rocks contain a great deal of information, and the task of a petrologist is to unravel and interpret that information. Traditionally, petrologic studies have been based on field work, often involving mapping to learn the field relationships between different kinds of rocks. During the last few decades, however, much petrologic research has moved indoors, into labs. Yet even petrologists who spend their entire careers in laboratories are studying materials that relate directly to natural materials and thus to a better understanding of planet Earth.

Petrologists may begin by examining rocks in outcrops. Figure 1.2 shows a geologist pointing at a large feldspar crystal in a granite outcrop in the Mojave Desert, California. The presence of both large and small mineral grains in this rock suggests it crystallized in two stages. We can see many rock characteristics, such as grain size, with the naked eye, or sometimes with the aid of a hand lens, while examining outcrops. Seeing other characteristics can require more sophisticated equipment or special specimen preparation, so geologists frequently break off and collect specimens to take back to laboratories for further study.
When studying rocks, some key considerations are rock compositions and the minerals present, textural relationships between minerals and other rock components, and larger-scale relationships between rocks of different sorts. So, petrologic research is a hybrid science that involves aspects of chemistry, mineralogy, physics, geological mapping, and sometimes biology. Because petrology is such a broad discipline, different petrologists develop expertise in different areas.

Besides investigating the origins of specific rocks or specific geological terranes, petrology also involves a bigger picture: the nature and evolution of Earth as a whole – in particular its surficial features, the deeper crust, and the mantle. The petrology at Earth’s surface is particularly important, because we interact with it every day. So, for example, petrology may help determine which areas are appropriate for agriculture. Figure 1.3 shows sugar cane growing on very young Hawaiian soils derived from weathered basalt. Even very young basalt can support robust cane growth.

Petrology is also important because it is the key to discovery and development of mineral resources. Ore deposits are intimately associated with rocks, so understanding rocks guides mineral exploration and development. For example, Figure 1.4 shows ore rock from the Stillwater igneous complex in Montana. The shiny minerals present are pyrite (gold-colored) and pyrrhotite (slightly less golden than the pyrite), and both minerals contain valuable amounts of the metals platinum and palladium. Petrologic investigations at Stillwater have allowed miners to extend mining operations so the Stillwater Mine is the number one platinum and palladium producer in North America.
Besides direct uses, fundamental principles and lessons learned from petrology have applications in modern industry. Metallurgy and crystallography, for example, began with studies of rocks and minerals. Today the lessons learned apply to a wide variety of synthetic minerals. Crystallography is especially important because it is a key that led to the creation of microchips and modern electronics.
1.1.2 Petrography
The field of petrography, really a subdiscipline of petrology, deals specifically with the description and classification of rocks. Most petrologic research involves petrography, typically involving examination of rocks in outcrop and hand sample, but often most importantly by examining rocks at high magnification.

High magnification can reveal things not seen otherwise, so petrologists often study rocks by looking at thin sections – thin slices of rock glued to a glass slide – using a polarizing petrographic microscope, such as the one seen in Figure 1.5. Petrographic microscopes are similar in most ways to other types of microscopes, except that the light that comes from below the stage passes through a polarizing filter. We can insert a second polarizing filter in the column above the stage when wanted. The filters allow us to see rock and mineral properties that we could not see otherwise. Petrographic microscopes reveal details that permit accurate mineral identification and, often, reveal information about rock crystallization history. Petrologists use rock textures and mineral relationships to interpret rock petrogenesis – the origin and nature of the processes that created a rock.

The top photo in Figure 1.6 shows a typical thin section. A slice of rock, glued to the glass slide, is so thin (30 μm) that light can pass through most mineral grains. Still, some grains in the thin section show various hues of green to black. Mineral colors may become more pronounced when viewed at high magnification. For example, the bottom photo in Figure 1.6 is a photograph of a thin section of granite, viewed with a petrographic microscope. Light from beneath the thin section passes easily through quartz (qtz), plagioclase (pl) and K-feldspar (kfs) grains, so they appear white – although grain boundaries are visible. The biotite (bt) and sphene (sph), however, appear darker and colored because the very thin mineral grains only allow specific wavelengths (colors) of light to pass through them.
Mineral colors in thin section do not typically match the colors seen when examining a larger hand specimen. This is because the color of a hand specimen is the color of light reflected from the specimen’s surface, while the color seen in thin section is the color that is transmitted by light passing through the rock slice.
Chapter 16 of this book contains a photo gallery with many photos of rocks in thin section.
For a more detailed discussion of optical theory and optical mineralogy, go to https://opengeology.org/Mineralogy/5-optical-mineralogy/
1.1.3 Geochemistry
Studying rocks to learn about Earth’s evolution, the distribution of natural resources, the nature of volcanic hazards, and many other things, requires understanding how different rocks form in different environments. Often, especially for igneous rocks, we gain the most important insights by examining rock (and magma) compositions. Thus, many petrologists study igneous rocks by studying rock and magma chemistry. Although samples of (hot) lavas flowing at Earth’s surface are occasionally collected and analyzed, most geochemical studies of igneous rocks involve analyzing rocks long after they crystallized from magmas. Most geochemical investigations of sedimentary and metamorphic rocks, too, take place long after the rocks formed.

The most widely used method for rock analysis is X-ray Fluorescence Spectroscopy (XRF), and consequently XRF spectrometers are standard instruments in many geochemistry labs. Figure 1.7 shows a typical XRF machine. It has a small sample port on top, and a set of samples in black holders in the sample changer in front. Inside the XRF machine, an X-ray beam strikes a sample, causing atoms within the sample to fluoresce and emit X-radiation. Different elements emit characteristic secondary X-rays of different wavelengths, and the intensities at each wavelength are proportional to the amount of the element present. XRF analysis is quite rapid compared with most other analytical techniques.
XRFs measure the concentration of elements ranging from major elements down to those present at the parts per million (ppm, equivalent to 0.0001 weight %) level. For low concentrations, however, other analytical techniques have lower detection limits and better precision. Another disadvantage of XRFs is that it cannot easily analyze sodium and lighter elements. The instrument, however, can analyze most elements with atomic numbers between 11 and 41 (sodium-niobium) and some heavier ones.
A second, widely used analytical technique is neutron activation analysis. There are several kinds of neutron activation analysis, but the most common is instrumental neutron activation analysis (INAA). To obtain an INAA analysis, a beam of neutrons is focused on a sample. The neutrons convert elements present to radioactive nuclides associated with the specific elements. Once samples are activated, the radioactive specimens “cool” for a short time, to let the most radioactive elements decay. Then they are ready for analysis. INAA analyses cannot be done in a standard geochemistry laboratory because the source of the neutron beam must be a (research) nuclear reactor; several different ones are available at sites in the United States.
As radioactive nuclides decay, they emit gamma rays with wavelengths characteristic of the elements present and intensities proportional to the amounts present. Some nuclides decay faster than others do, some decay quite slowly, and there are other complications due to overlapping wavelengths. So, measuring gamma ray intensities to obtain analyses is not quickly done. Initial analyses generally take place four or five days after activation and then again a month later. Some elements require measurements a year after activation, although many researchers do not want to wait that long and, thus, will not analyze elements such as gadolinium (Gd). INAA is an especially good method for analyzing rare earth, platinum group, and a few other important elements.

Inductively coupled plasma mass spectrometry (ICP-MS) is a third excellent way to obtain analyses. Figure 1.8 is a photo of a machine used for this purpose. Sample preparation is more complicated than for XRF or INAA analyses, because samples must be dissolved, which requires time and caustic acids. Once the samples are in solution, the liquid passes through a plasma torch. The flame emits light having wavelengths particular to the elements present and, conventionally, light intensity is used to learn elemental concentration (ICP). More significantly, today, we can send emitted ions to a mass spectrometer (MS), allowing more precise measurement and greater sensitivity when analyzing elements present in very small amounts. ICP-MS also allows measurement of the concentrations of specific isotopes. Although primarily used for trace elements and isotopes, some variations of ICP-MS permit analyses of major elements, too.
Petrologists also sometimes use other techniques for element and isotope analysis. All analytical techniques have advantages and disadvantages, and each technique can analyze a specific group of elements (or isotopes) over specific ranges of concentration. For the most thorough characterization, many different methods should be combined, but this is rarely possible. So, depending on purpose, one or two techniques are typically chosen, often depending on the instrumentation that is locally available to the researcher. Consequently, some studies report a different number of elements than others do. Furthermore, some elements are especially problematic due to extremely low concentrations or for other reasons and so rarely analyzed. Most commonly today, researchers use a combination of XRF and INAA to get a relatively complete chemical characterization of a rock sample.
1.2 Three Kinds of Rocks
Although there is occasional ambiguity, we commonly divide rocks into three classes: igneous rocks, sedimentary rocks, and metamorphic rocks. These three different kinds of rocks form by distinctly different process as shown in Figure 1.9.

1.2.1 Igneous Rocks
Igneous rocks are created when molten rock (magma) cools and hardens. The result is sometimes a crystalline rock composed entirely of visible mineral crystals. But some igneous rocks, especially those that form from lavas (magmas that flow on Earth’s surface) are so fine grained that mineral crystals, even if present, cannot be seen. Figure 1.10, for example, shows a rock that contains no crystals; it is entirely glass. Other igneous rocks are partly to entirely volcanic glass that is not considered a mineral (because it is not crystalline). Many volcanic rocks contain combinations of crystals and glass, but some are entirely glass; if so, we call them obsidian. Figure 1.10 shows an example of obsidian from near Redmond, Oregon.
Igneous rocks, like rocks of other sorts, may contain a single component (for example the volcanic glass in Figure 1.10) or multiple components. The rock in Figure 1.11, for example, is a granite from Bedretto, Switzerland. It contains several different minerals. White feldspar, grayish quartz, and black biotite are most obvious in this photo. And, the photo in Figure 1.12 shows a granitic dike that contains the same minerals, cutting through a gneiss (a metamorphic rock). Many igneous rocks show little structure left over from when the first formed, and they may have textures/structures that are the same in all directions. Still, sometimes, as in Figure 1.12, igneous rocks have shapes or textures indicative of flowing magma and an intrusive origin.
1.2.2 Sedimentary Rocks
Sedimentary rocks fall into several categories. Most sedimentary rocks, called clastic rocks, contain individual clasts that are mineral crystals and, perhaps, fragments of other rocks, or fossils or other organic materials, that have become compressed and cemented together. We call the process that creates clastic sedimentary rocks in this way lithification. Lithification typically involves compression and cementation during burial. It may also involve recrystallization as individual mineral grains combine to make larger crystals.
Figures 1.13, 1.14, and 1.15 show three examples of clastic rocks. The red sandstone in Figure 1.13 is mostly quartz stained by iron oxide, but it also contains clasts of other sorts. The shale in Figure 1.14 consists of fine-grained clay minerals too small to see. And the conglomerate in Figure 1.15 is mostly rounded clasts of rock cemented together with a finer-grained matrix between the clasts.
Other sedimentary rocks, called chemical sedimentary rocks, form when minerals precipitate from water, sometimes partly or entirely through biological processes. The photo in Figure 1.16 shows one of the largest salt mines in Europe. The rock in the photo is entirely halite (table salt) that precipitated from seawater. Still other sedimentary rocks, called organic sedimentary rocks, such as coal, form entirely from biological debris. Figure 1.17 shows an example – bituminous coal from Ohio. And, some sedimentary rocks are combinations of clasts and precipitated materials and so are part way between being clastic and chemical rocks.
The photos below show examples of limestone. The limestone in Figure 1.18 consists entirely of the mineral calcite. The rock in Figure 1.19 is another limestone, but it contains fossils as well as calcite. Limestones are typically clastic rocks formed from organic remains, but sometimes they form by precipitation from water, or by organic processes. Distinguishing the different origins can be difficult.
Gravity deposits clastic sediments in horizontal layers that may cover vast regions. Chemical sediments are typically deposited in layers, too, perhaps at the bottom of an ocean. Consequently, sedimentary rocks often are in thick horizontal rock units, or beds, with relatively uniform composition. Individual beds may be centimeters to meters thick, and similar beds commonly form one above another. Figure 1.20 shows beds of sedimentary rocks in the Grand Canyon. These rock formations derived from sediments deposited over a time span of about 350 million years. The different layers of sedimentary rocks include siltstone, sandstone, shale, and limestone. Most of the cliffs are made of limestone because it is more erosion resistant than the other lithologies. The slopes are made of shale.
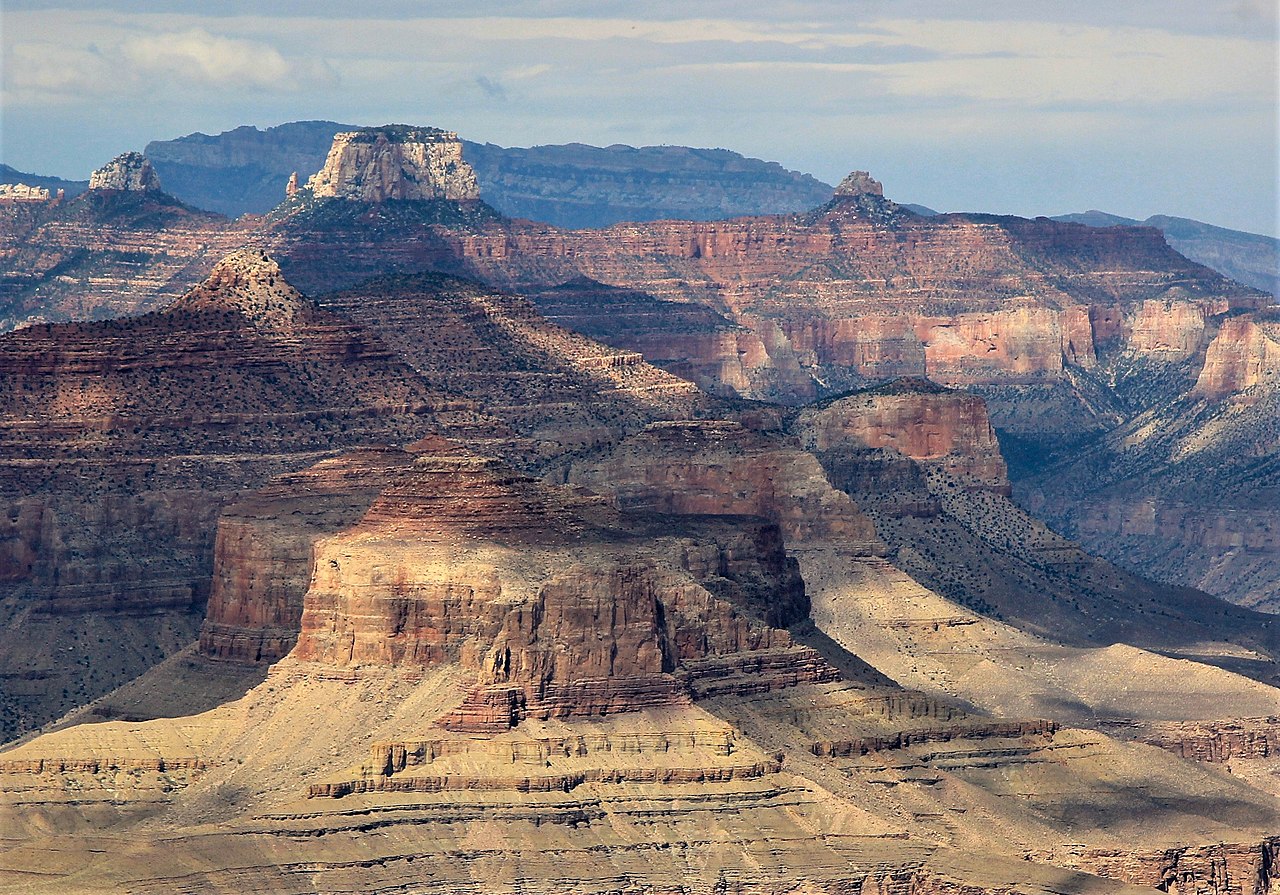
1.2.3 Metamorphic Rocks
Metamorphic rocks form by alteration or modification of any kind of preexisting rock (Figure 1.9). Metamorphism may be caused by pressure, heat, or by water or other fluids or gases that infiltrate a protolith. The process of metamorphism can involve changes in the minerals present, changes in rock texture, or changes in rock composition, or any combination of the three. We call the original rock the protolith, and the composition of the protolith limits the kind of metamorphic rock that can form. The amount of change during metamorphism generally depends on temperature, which can range from Earth-surface temperatures to the much greater temperatures experienced by rocks at depth within Earth. Although temperatures of metamorphism may be quite high, by definition, metamorphism does not involve melting.
The photos below show some metamorphic rocks. Figure 1.21 is an example of quartzite. It contains only quartz but the quartz grains, which once were separate grains in a sandstone, have grown together. The photo in Figure 1.22 is a garnet-chlorite schist from Alabama that formed when shale was metamorphosed. The red equant garnets and the green chlorite are easily distinguished. The photo in Figure 1.23 is a type of high-pressure rock called a blueschist that formed by metamorphism of basalt. Blue amphibole makes up most of the rock, but some red garnet and green pyroxene are also present.
|
|
During metamorphism, pressure on a rock may not be the same in all directions. Consequently, metamorphic rocks may develop foliations – a layered appearance due to parallel alignment of mineral crystals, or due to layers of different mineral composition. Figures 1.24 and 1.25 show two examples. The talc schist in Figure 1.24 is composed almost entirely of talc crystals that have aligned parallel to give the rock a layered flaky foliation characteristic of schists. Most schists are mica schists. Aligned micas, most commonly biotite or muscovite instead of talc, give most schists their texture. The rock in Figure 1.25 is a gneiss, a high-temperature kind of metamorphic rock. Gneisses contain alternating bands of minerals of different colors, typically white or red alternating with gray and black.
|
1.3 The Rock Cycle
Examination of Figure 1.9 reveals that any preexisting rock (igneous, sedimentary or metamorphic) may melt to become magma and, thus, produce an igneous rock. Similarly, any preexisting rock may weather to produce sediment that eventually becomes a sedimentary rock. And any preexisting rock may be metamorphosed. These observations give rise to the concept of the rock cycle – the notion that rocks and minerals cycle between igneous, sedimentary, and metamorphic pedigrees. The rock cycle is commonly talked about in introductory geology texts but the notion is quite misleading because few rocks actually cycle as implied – changing from one kind to another and another in an ongoing process. The key point, however, is that geological materials do change and evolve over the immensity of geologic time, and rocks may occasionally switch from one class (igneous, sedimentary, and metamorphic) to another.
1.4 Scope of this Book
In this book, we are going to focus only on igneous and metamorphic petrology; we will say no more about sedimentary petrology. Igneous and metamorphic rocks go together well because they are often found together. And studies of both involve consideration of rock chemistry, thermodynamics, and phase diagrams. Sedimentary rocks are a different story. Variations in rock chemistry are less important, and phase diagrams do not apply because sedimentary rocks (because of low temperatures) generally do not reach chemical equilibrium. Furthermore, it is difficult to discuss sedimentary rocks without also discussing environments of deposition, stratigraphy, paleontology, tectonics, Earth history, and other things. So, sedimentary petrology deserves a separate book all on its own. We will begin our investigation of igneous and metamorphic rocks by looking at igneous rocks and the things that interest igneous petrologists.
● Figure CreditsUncredited graphics/photos came from the authors and other primary contributors to this book. 1.0 (opening photo) Basalt with olivine, incidencematrix, Wikimedia Commons |