
KEY CONCEPTS
- Metapelites are metamorphosed clay-rich sedimentary rocks.
- Different pelitic index minerals form at different grades of metamorphism.
- Isograds, based on index minerals, are lines on maps that separate zones of different metamorphic grades.
- Different characteristic minerals develop in metapelites that form at high, medium, and low pressure.
- Most key reactions that occur during pelite metamorphism are dehydration reactions.
- At high temperature, metapelites may melt before reaching granulite-facies metamorphic conditions.
- We commonly show metapelitic minerals and assemblages on AFM diagrams.
14.1 Metamorphic Grade and Pelitic Rocks
14.1.1 Index Minerals
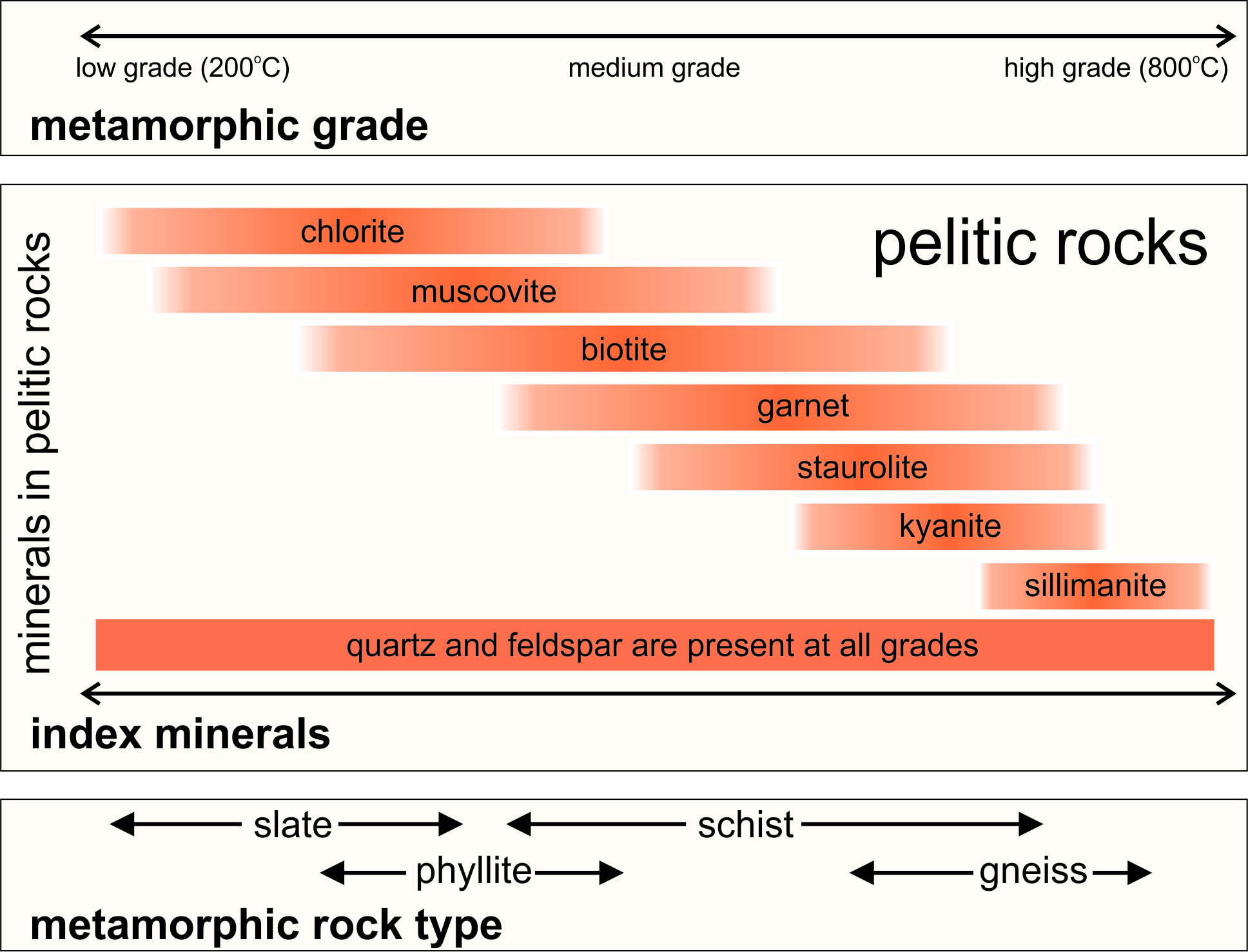
We use the general term pelite to refer to fine-grained clay-rich sedimentary rocks such as shale or mudstone. When metamorphosed, we call these rocks metapelites. Low-grade metapelites form at relatively low temperature (200-400 oC), medium grade metapelites at medium temperatures (350-650 oC), and high-grade metapelites at high temperatures (>600 oC).
Metapelites have a number of common appearances. Generally, they develop foliations to become slates, phyllites, schists or gneisses. The bottom part of Figure 14.1 shows how different kinds of foliations correlate with grade.
Metamorphism also produces distinctive index minerals that form at different metamorphic grades. Figure 14.1 shows the most important index minerals from low grade to high: chlorite, muscovite, biotite, garnet, staurolite, kyanite, and sillimanite. The sequence of minerals depicted in Figure 14.1 is typical for metapelites undergoing Barrovian metamorphism. But there is variation depending on rock composition. Additionally, different index minerals may form during contact or low-pressure metamorphism, or during high-pressure metamorphism.
The significance of index minerals was first pointed out by Scottish geologist George Barrow in his classic 1912 study of regionally metamorphosed pelitic rocks in the Scottish Highlands. Barrow showed that during normal progressive metamorphism, pelites start unmetamorphosed and the index minerals appear in predicable order as grade increases. So, the most common way to estimate the metamorphic grade of pelitic rocks is based on the presence or absence of the minerals seen in Figure 14.1.
Table 14.1 Common Metamorphic Minerals in Pelitic Rocks | ||
grade | minerals | formulas |
low grade![]() |
chlorite muscovite biotite garnet staurolite kyanite sillimanite |
(Mg,Fe)5Al2Si3O10(OH)8 KAl2(AlSi3)O10(OH)2 K(Fe,Mg)3(AlSi3)O10(OH)2 (Fe,Ca,Mg)3Al2Si3O12 Fe2Al9Si4O22(O,OH)2 Al2SiO5 Al2SiO5 |
Table 14.1 lists compositions for the pelitic index minerals. In contrast with mafic and most other kinds of rocks, metapelites are rich in aluminum, and all of the index minerals are aluminosilicates. Besides those listed, other aluminous minerals, including chloritoid and andalusite, are sometimes considered index minerals, too. The abundance of aluminous minerals in metapelites is because the dominant minerals (60% or more) in pelite protoliths (shales) are clay minerals, all of which contain aluminum.
Chlorite is found only in low-grade pelitic rocks that often show little metamorphic texture other than, perhaps, slaty cleavage. With increasing metamorphism, muscovite and biotite appear, and rocks may be phyllites or schists. At still higher grades, garnet, staurolite, kyanite, and sillimanite can appear. Quartz and feldspar are present in rocks of all grades. At the highest grades, micas are absent and rocks are typically gneisses. One of the Al2SiO5 polymorphs (kyanite, andalusite, or sillimanite) may be present in rocks of all grades, but need not be.

Figure 14.2 (a Thompson AFM projection) shows, in red, the general composition range for pelitic rocks. Some pelites, termed high-Al pelites, contain more aluminum than others. Highly aluminous minerals, including any of the Al2SiO5 minerals, staurolite, chloritoid, and cordierite, are common in high-Al pelites. They are minor or absent from low-Al pelites. Nonetheless, different minerals reflect variations in metamorphic grade and, if present, form in the same order during progressive metamorphism, no matter rock composition.
Figure 14.2 also shows, in blue, the compositions of average granites. Granites have similar compositions to pelites, except granites have substantially less Al2O3. Generally metagranites contain K-feldspar, quartz, and biotite – and none of the Al-rich minerals in the diagram. Occasionally, however, metagranites contain enough aluminum so that some of the other minerals may be present.
● Box 14.1 Minerals, Abbreviations and FormulasThe table below lists minerals, their formulas, and abbreviations used in figures and some other places in the rest of this chapter.
|
14.1.2 Some Constraints on Pelite Metamorphism

Most pelitic metamorphism occurs at pressures less than 14 or 15 kbar and temperatures less than 800 oC (Figure 14.3). On a coarse scale, we can divide this P-T space into regions where different Al2SiO5 minerals (kyanite, andalusite, or sillimanite) are stable. When present, these aluminosilicate minerals, then, tell us whether a rock equilibrated at relatively high pressure (kyanite), low pressure (andalusite), or at high temperature (sillimanite). We can also divide the diagram based on the presence or absence of muscovite. Most metapelites contain muscovite. However, muscovite breaks down and is absent from pelites that equilibrate at the highest temperatures where conditions move into the granulite facies. The blue reaction line shows where the “muscovite-out” reaction occurs. If a metapelite contains muscovite, metamorphism must have occurred in the white region at temperatures below this curve.
Figure 14.3 also shows the melting curve (dashed black line) for an average water-saturated pelite. Melting may occur at temperatures as low as 650 oC, depending on pressure. So, if a pelite contains H2O, it will likely melt before metamorphic temperatures can reach the high-grade conditions on the right side of the diagram. This happens even if the rock contains only a few percent H2O. However if a rock is “dry” (contains no H2O), melting temperature may be in excess of 1,000 oC , and very high-temperature metamorphic rocks may form. Mafic rocks, even when saturated with water, melt at significantly higher temperatures than pelites. Consequently, high-temperature metabasites are more common than high-temperature metapelites.
14.1.3 Different Facies Series
The series of minerals depicted in Figure 14.1 and Table 14.1 is the Barrovian Series, named in honor of George Barrow. These minerals form in pelitic rocks when metamorphism occurs at conditions on a Barrovian P-T path, shown by a black arrow in Figure 14.3. (See also Figure 13.4, and the discussion of metabasites and facies series in Chapter 13). Barrovian metamorphism is the most common kind of metamorphism.

In the Buchan region of Scotland, just 100-150 kilometers northeast of where Barrow did his classic study, metamorphism occurred at pressures lower than normal Barrovian metamorphism. This kind of metamorphism, Buchan metamorphism, occurs at conditions on the lower black arrow in Figure 14.3. Buchan metamorphism produces many of the same minerals that Barrovian metamorphism produces. However, cordierite and andalusite, low-pressure minerals that do not generally form during Barrovian metamorphism, are often present. Additionally, the low pressures of Buchan metamorphism mean that kyanite is absent. Figure 14.4 is a photo of an andalusite-cordierite schist from the Buchan terrane.
14.2 Mapping Regional Metamorphic Terranes
The temperatures and pressures at which rocks equilibrated typically vary across a geological terrane (thus giving rise to metamorphic field gradients and facies series). Consequently, for rocks of the same composition, different mineral assemblages may be found in different places. These differences may be due to regional variations in metamorphic conditions at the time that metamorphism occurred. Alternatively, metamorphic conditions may have been the same across a region, and variations in mineralogies reflect different amounts of uplift and erosion that uncovered rocks from different depths. It can be difficult to distinguish the two possibilities and, in some places, it is clear that both have had an effect.

When petrologists study regional metamorphism, a fundamental undertaking is to document any regional variations in metamorphism by mapping metamorphic zones. These zones are regions, small to large, characterized by specific index minerals. Figure 14.5 is based on one of the first maps of metamorphic zones, created by George Barrow in 1918. The dot on the inset map shows where in Scotland Barrow made this map. In this region, called the Dalradian Region, unmetamorphosed rocks to the south and east of the Highland Boundary fault (which follows the southern edge of the chlorite zone on the map) give way to zones containing chlorite, biotite, garnet, staurolite, kyanite, and sillimanite to the northwest. These are all pelitic index minerals, and they demonstrate that metamorphic grade increases to the north and west (red arrow).
To make a map such as the one shown above, geologists visit outcrops in the field to find where different index minerals occur. They then can draw isograds on a map – lines that separate zones of different metamorphic grade. We generally name isograds for the first appearance of a mineral with increasing grade. So, for example the biotite isograd, is the line on a map that separates areas containing biotite-free rocks from higher-grade areas with rocks that contain biotite. And, the garnet isograd is the line that separates regions of lower-grade rocks from higher-grade rocks that contain garnet. Mapping zones and isograds is more easily done for metapelites than for other kinds of rocks. This is because pelitic index minerals are often easily identified, the reactions that create them may produce sharp zone boundaries, and also because there are half a dozen or so pelitic index minerals that may be present in any region.
The isograds drawn on the map in Figure 14.5 suggest that zone boundaries can be precisely mapped. Often, however, isograds are interpretations based on limited evidence, in large part because finding outcrops containing key minerals may be challenging. The presence or absence of index minerals depends on rock composition, not just on metamorphic grade. Additionally, many metamorphic terranes contain few outcrops to examine.
Table 14.3 compares metamorphic facies (defined by assemblages in metabasites) with different pelitic metamorphic zones. The chlorite and biotite zones correspond to the greenschist faces. With increasing grade, metamorphism moves into the amphibolite facies as garnet, staurolite, kyanite, and sillimanite appear. In pelitic rocks, sillimanite with K-feldspar is generally recognized as representing granulite facies conditions. Note that, as implied by Table 14.3, mineral assemblages in metabasites are highly variable and different zones may be characterized by several different assemblages. This is because mafic minerals have quite variable compositions, and the reactions that relate the minerals are continuous.
Table 14.3 Comparing Metamorphic Facies and Metamorphic Zones | ||
facies | pelitic zone | minerals in metabasites |
greenschist | chlorite biotite |
chlorite, albite, epidote, ± actinolite, ± calcite |
amphibolite | garnet staurolite kyanite sillimanite |
hornblende, plagioclase, ± epidote, ± garnet, ± clinopyroxene |
granulite | sillimanite + K-feldspar | orthopyroxene, clinopyroxene, plagioclase, quartz, ± garnet, ± hornblende, ± biotite |
Chapter 16 Section 16.3.4 contains many photos of metamorphosed mafic and ultramafic rocks in hand specimen and in thin section. If you want to know what they look like, go there. |
14.3 Petrogenetic Grid for Metapelites
In contrast with metabasites, many important reactions in metapelites are discontinuous or nearly so (being continuous over a relatively small range of temperature). Reactions may be plotted as lines on P-T diagrams, permitting construction of petrogenetic grids such as the one in Figure 14.6 (below). This phase diagram was calculated for a model system that contains the chemical components listed at its top. These are the most important components of metapelitic rocks.

The reactions in the phase diagram above are not balanced, and to avoid clutter, we have omitted quartz and H2O from the reaction labels (although these phases are involved in most of the reactions). Essentially, for simplicity we have projected the reactions from quartz and H2O onto the P-T diagram, which is tantamount to assuming that quartz and H2O are present in the rocks being considered. Figure 14.7 contains AFM diagrams showing stable mineral assemblages for P-T conditions in each of the lettered zones in the phase diagram above. The vertical lines between the triangles depict the reactions that are crossed moving from one lettered zone to the next.
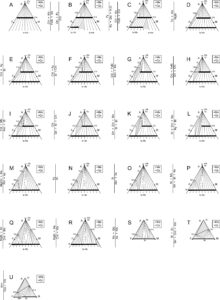
● Box 14.2 Interpreting AFM Diagrams for Metapelites![]() The AFM diagrams in this chapter are Thompson projections. In these diagrams, shaded regions show 3-phase assemblages, white regions with subparallel lines show 2-phase regions, dots show singular mineral compositions, and black lines show binary mineral solutions. Projected phases are in a box up and to the right of the diagrams – these phases are assumed to be present when we use AFM diagrams. For more detailed discussion of how AFM diagrams are made and interpreted, go to Section 11.5.4 in Chapter 11. |
The petrogenetic grid in Figure 14.6 shows reactions that can occur during metamorphism of pelites. So, we can predict the minerals and assemblages that will be stable under different P-T conditions. Conversely, if we have a rock containing a particular assemblage, we can use the grid to estimate the conditions at which it equilibrated. However, interpreting complex phase diagrams with many reaction can be challenging. And some of the reactions in Figure 14.6 only apply to some mineral compositions. We avoid some interpretation problems by using the AFM diagrams in Figure 14.7.
If we want to know the pressure-temperature conditions where a particular assemblage is stable, we can look at the AFM diagrams to see which show that the assemblage is possible. Because each AFM triangle corresponds to a lettered zone on the phase diagram, we then know where in P-T space the assemblage is stable. This approach is best explained by example.
Consider three rocks containing hypothetical assemblages, one low-grade (assemblage 1), one medium grade (assemblage 2), and one high grade (assemblage 3):
space 1: chloritoid + chlorite + pyrophyllite + muscovite + quartz
space 2: garnet + chlorite + muscovite + quartz
space 3: garnet + biotite + sillimanite + muscovite + quartz

Assemblage 1 is stable if choritoid, chlorite, and pyrophyllite are stable together. Examination of Figure 14.7 reveals that only AFM diagram B contains a triangle connecting these minerals. This means that assemblage formed at conditions shown by the blue region on the left in Figure 14.9
Assemblage 2 is stable if garnet and chlorite are stable together. A tie-line connects garnet and chlorite in AFM diagrams H, I, and J – nowhere else. This corresponds to the middle blue region in Figure 14.9.
Assemblage 3 is only stable if garnet, biotite, and sillimanite are stable together. Triangles connecting these minerals are found in AFM diagrams P, Q, R, and S. But muscovite is not stable in AFM triangle S. So this assemblage must equilibrate in the large blue region on the right in Figure 14.9
14.4 Barrovian Metamorphism

The phase diagram in Figure 14.10 is the same as the one seen previously, except that the path of Barrovian metamorphism is shown with a red arrow. Low-temperature regions B, C, and part of D are equivalent to the greenschist facies conditions described by Eskola. Zones E through R cover amphibolite facies conditions, and zones S and T are equivalent to the low-pressure part of the granulite facies. So, Barrovian metamorphism includes the greenschist, amphibolite, and granulite facies. Below we consider the evolution of rocks, and development of different index minerals, for pelitic rocks that follow the normal Barrovian path of metamorphism (red arrow).
14.4.1 The Biotite Isograd

Pelitic protoliths contain mostly clay and quartz, perhaps with organic matter. A small amount of metamorphism may produce chlorite and the lowest-grade mappable zone is usually the chlorite zone. Quartz and remnant clay may be present in chlorite-grade rocks. With further heating, muscovite and perhaps pyrophyllite appear, and eventually chlorite and K-feldspar will react to produce biotite.

Figure 14.11 shows the Thompson projections illustrating the changes that occur as chlorite reacts to form chloritoid and biotite. AFM triangle 14.11A is for very low-temperature conditions where chlorite is the only stable AFM mineral. Triangle 14.11B depicts stable assemblages above the biotite isograd, corresponding to the blue region in the phase diagram in Figure 14.12.
The changes from AFM diagram A to diagram B in Figure 14.11 involve two reactions, shown by labeled vertical lines between the two triangles: Fe-rich chlorite reacts with K-feldspar and with pyrophyllite to produce biotite and chloritoid, thus crossing the biotite and chloritoid isograds. The chloritoid isograd, however, is seldom mapped in metamorphic terranes because chloritoid is relatively rare, only forming in especially Fe-rich pelites.


Chlorite, muscovite, and biotite schists are the most common kinds of metapelites. At lower grades – before micas develop – metapelites are generally very fine-grained and mineral identification is problematic. Although chlorite is often very fine-grained, micas, both muscovite and biotite, commonly form easily identified macroscopic crystals when they develop. Figure 14.13 shows a relatively low-grade muscovite schist, and Figure 14.14 is a biotite schist that formed when P-T conditions passed the biotite isograd.
14.4.2 The Garnet Isograd

Garnet can form by any of several reactions between about 500 and 600 oC. The reactions occur in the blue region in the phase diagram in Figure 14.15, and are shown by vertical lines between the triangles in the Thompson projections below (Figure 14.16). Garnet is further stabilized, and its stability range expands, in rocks that contain significant amounts of manganese but the phase diagrams and chemographic projections seen in this chapter do not take this into account. In normal Barrovian metamorphism, garnet forms at temperatures of 500 to 575 oC, and pressures around 6 kbar. At lower pressures where Buchan metamorphism occurs cordierite may be present instead of garnet.

Figure 14.16 shows Thompson projections for conditions equivalent to zones F and J in Figure 14.15. These zones represent conditions in the amphibolite facies that are below, and above, the garnet isograd. The biggest change between zones F and J is that chloritoid disappears and garnet appears in its place.
Any of the reactions shown by vertical lines in Figure 14.16 may produce garnet. The reaction or reactions that occur depend on the compositions of the reactants. Two of the reactions produce extremely almandine-rich garnet (with composition that plots near the A-F side of the triangle), and are labeled with Alm instead of Grt in Figure 14.16.
Figure 14.17 below is a photo of an outcrop of garnet schist near Custer, South Dakota. Figure 14.18 is a photo of garnet phyllite from the Tin Mountain Mine, also near Custer. This phyllite, like many phyllites, contains millimeter-sized garnet crystals. Figure 14.19 is a photo of a higher-grade garnet-biotite schist with coarser crystals, including a large euhedral garnet porphyroblast that is 3 cm across.



blank
14.4.3 The Staurolite, Kyanite, and Sillimanite Isograds
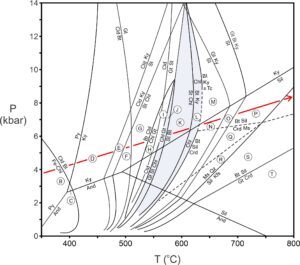
Although extremely Fe-rich staurolite is stable in some unusual composition rocks at temperatures as low as 500 oC, transition from zone J to K (Figure 14.20) generally marks the staurolite isograd. Figure 14.22 (below) shows chemographic diagrams for these zones.
Many changes occur between 550 oC and 650 oC during Barrovian metamorphism (blue region in Figure 14.20). Staurolite first appears at around 550 oC and 6 kbar. At slightly higher temperatures, in zone K, staurolite and biotite may coexist. And at about 600 oC, chlorite disappears and kyanite becomes stable with biotite (zone L). Eventually, when temperature reaches 65o oC or so, sillimanite replaces kyanite.
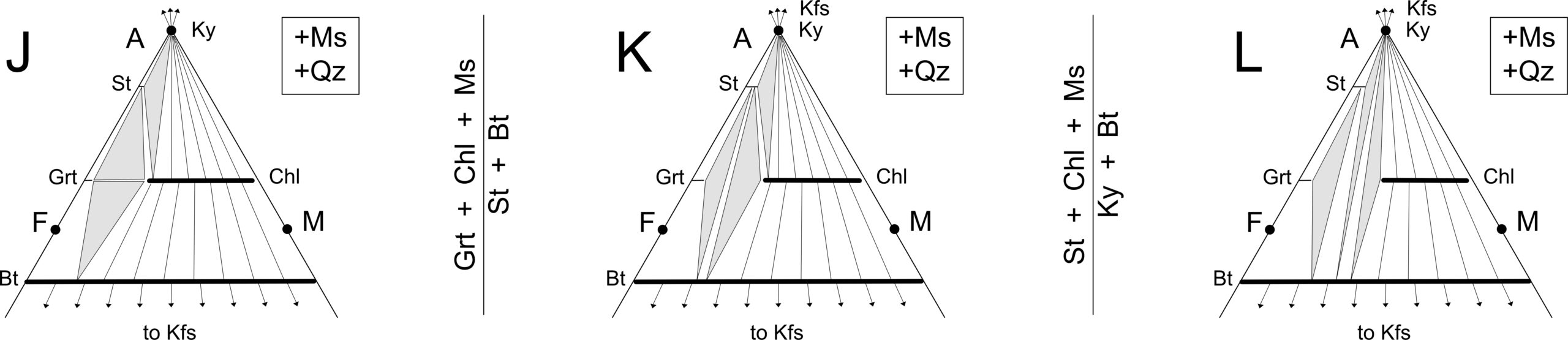
Figure 14.22 shows a biotite-staurolite schist from near Custer, South Dakota. Figure 14.23 is a garnet-staurolite schist from Vermont. Besides garnet and staurolite, this schist includes significant amounts of biotite, muscovite, chlorite, and quartz, as well as some other accessory minerals. However, most of these minerals can only be identified with a microscope. Figures 14.24 and 14.25 are two examples of kyanite schists. Some schists of this grade contain sillimanite instead of kyanite if they equilibrated at slightly lower pressures.
Figure 14.26 below is a rock composed almost entirely of sillimanite, silvery muscovite, and black biotite. The sillimanite in this rock is in the form of coarse blades, but sillimanite commonly is fine-grained and fibrous. Figure 14.27 is a sillimanite-garnet schist from the Coast Range of British Columbia. The sillimanite appears as long white needles embedded in quartz. Abundant biotite and feldspar are also present.
|
|
14.4.4 The Orthopyroxene Isograd

At very high temperatures, orthopyroxene may appear in metapelites as biotite breaks down. But, this is unusual because temperatures for this to occur exceed the melting points for pelites, unless they are exceptionally dry. If rocks are dry enough, and if temperature is high enough to stabilize orthopyroxene, other normally rare minerals may develop. These include sapphirine (Figure 14.28) and ossumilite. Sapphirine- and ossumilite-bearing rocks are, however, quite rare.
14.4.5 Minerals Out and the Granulite Facies
The preceding discussion was about the conditions at which different index minerals may form. But, minerals also disappear as metamorphism proceeds. For example, chloritoid is absent from zone J and higher-grade zones in the pelite phase diagram, and staurolite is absent from zone P and at higher grades. These minerals disappear because they dehydrate to produce more stable anhydrous mineral assemblages.
Figure 14.29 (below) shows the muscovite-out reaction. At temperatures above this curve, muscovite disappears by reaction with quartz:
space muscovite + quartz = K-feldspar + sillimanite + H2O,
In Figure 14.3, we highlighted this reaction (in blue) and noted that it occurs as conditions move into the granulite facies. However, the muscovite-out reaction is never reflected on normal Thompson AFM diagrams because we project from muscovite and quartz to create the diagrams.
|
|
Figure 14.30 is a slab of rock from the Adirondack Mountains that contains K-feldspar and sillimanite. Muscovite is absent, and this rock equilibrated at temperatures above the muscovite-out reaction. The rock also contains garnet and biotite. So, this rock equilibrated in Zone P of the pelite phase diagram we saw above.
The absence of muscovite from rocks metamorphosed at high grades, in the granulite facies, poses a problem because normal Thompson projections assume muscovite is present. However, if muscovite is absent because the muscovite out reaction occurred, K-feldspar will be present. So, Thompson’s solution was to project for K-feldspar instead of muscovite for rocks in which muscovite is absent. Figure 14.31 shows examples for zones S, T, and U of the pelite phase diagram we have seen previously. These AFM diagrams are quite similar to diagrams projected from muscovite, but biotite plots very near the FM base of the triangle in these projections.
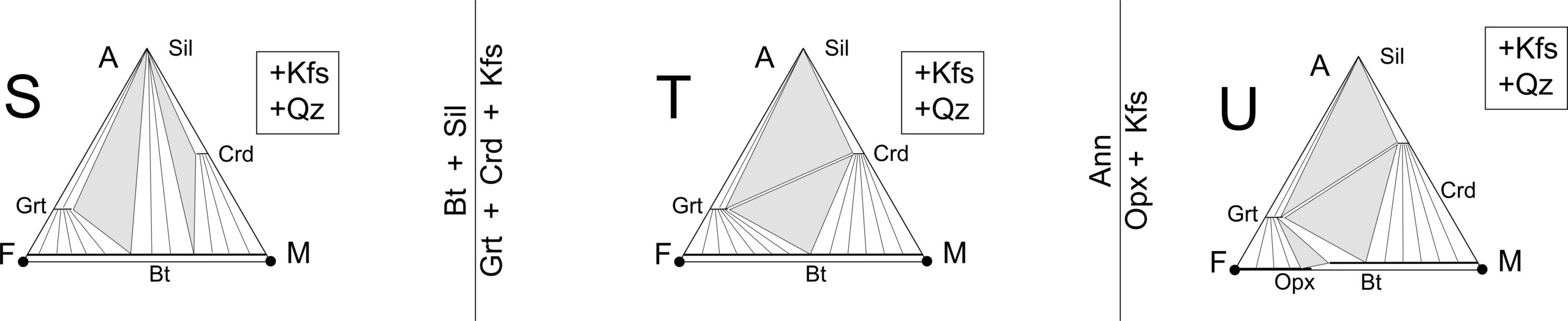
Moving from zone S to zone T (Figure 14.31) stabilizes garnet-cordierite assemblages. The rock in Figure 14.32 shows an example – an outcrop of garnet-cordierite gneiss in Namaqualand, South Africa. In this granulite-facies rock, finer-grained blue cordierite accompanies large rusty red garnet porphyroblasts.

Diagram U in Figure 14.31 shows stable orthopyroxene. This, as pointed out previously, is an uncommon occurrence, because unless they are dry, most pelites melt before temperatures become high enough to stabilize orthopyroxene.
14.5 Metamorphism of Pelites at High Pressure and Low Temperature

High-pressure metamorphism, involving the prehnite-pumpellyite, blueschist, and eclogite facies, is generally associated with subduction zones (blue arrow in Figure 14.33). By far, the most common rocks involved are mafic or ultramafic (because these rock make up the oceanic lithosphere), but pelites are sometime present.
Blueschist-facies metapelites often contain garnet, chloritoid, epidote, and kyanite. Perhaps the most important mineral distinctions are that plagioclase is absent and muscovite gains Mg and Fe to become phengite. Lawsonite, Na-rich amphibole (glaucophane or riebeckite) and Na-pyroxene (jadeite or aegirine) are also common. Some blueschist metapelites contain talc, paragonite, or stilpnomelane. Still others contain unusual minerals such as sudoite (a member of the chlorite group) and carpholite (a rare manganese silicate).
14.6 Metamorphism of Pelites at Low Pressure

Metamorphism of pelites at low pressure involves the facies highlighted in Figure 14.34. Low-pressure metamorphism is different from metamorphism at higher pressure in several ways. For example, andalusite and sillimanite are present in low-pressure rocks (instead of kyanite), cordierite is widespread and common, and garnet is absent. Additionally, because most of the reactions affecting pelites have positive P-T slopes, reactions at low pressure occur at lower temperatures than in higher-pressure rocks. So, chlorite, staurolite, and muscovite disappear at lower temperatures than they do during Barrovian or Buchan metamorphism.
14.6.1 Hornfels Facies
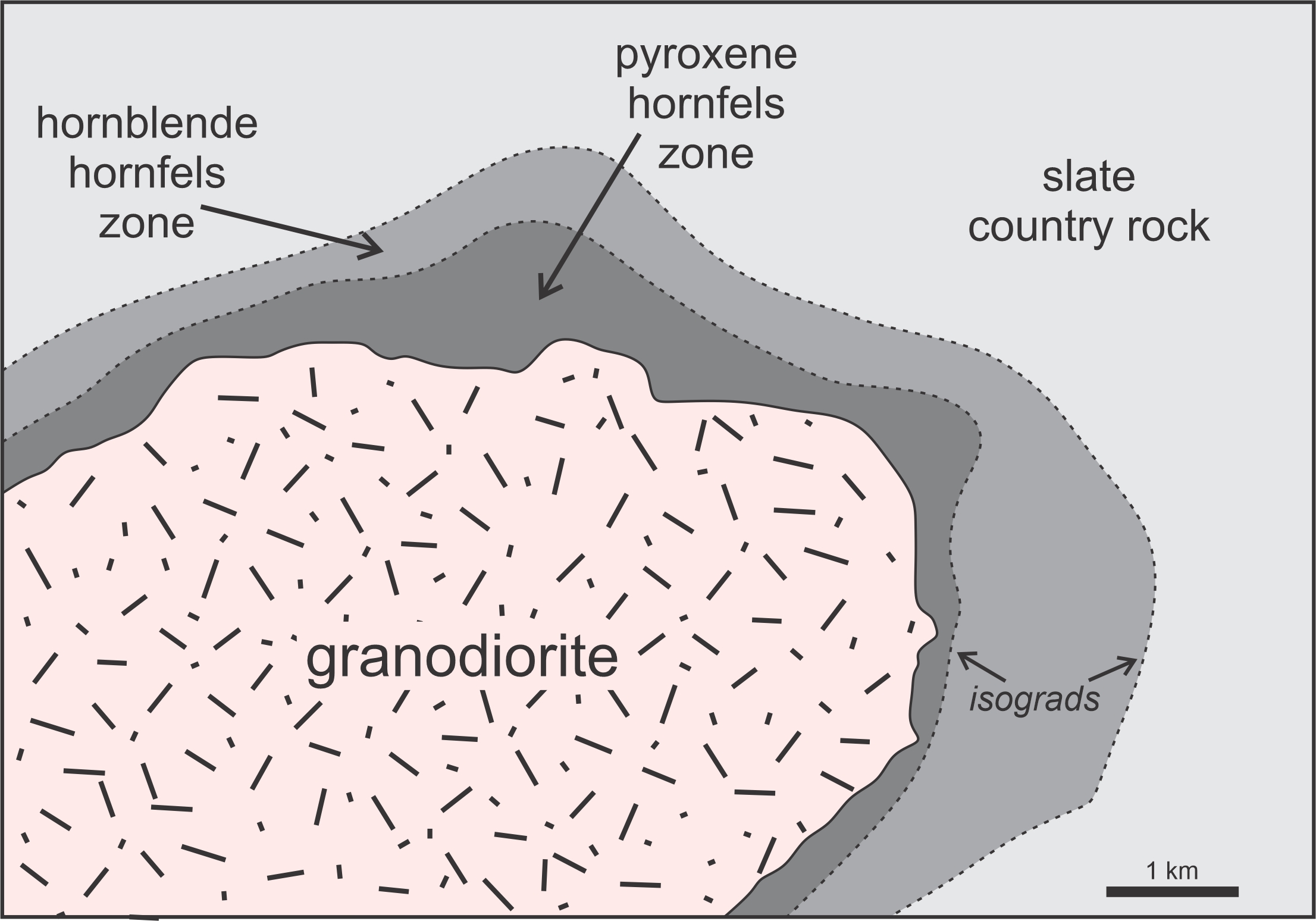
Low pressure is the realm of contact metamorphism. Figure 14.35 is a map of a contact aureole around the Devonian Onawa Pluton, a granodiorite body in central Maine. The pluton, an elliptical granodiorite body, intruded into a slate country rock. The slate was a product of earlier regional metamorphism that occurred before the Onawa pluton formed. Heat from the intrusion created a contact aureole that is up to 2 km thick on some sides of the pluton. Studies by Moore (1960) and Symmes and Ferry (1995) determined that this aureole formed at about 3 kbar pressure and a maximum temperature of about 650 oC.

The Onawa aureole contains two distinct metamorphic zones between the granodiorite intrusion and the country rock. The outer zone was metamorphosed in the hornblende-hornfels subdivision of the hornfels facies (shown in Figure 14.34). Rocks there contain biotite, andalusite, cordierite, muscovite, quartz, and plagioclase. The majority of rocks have a spotted appearance, like the hornfels in Figure 14.36. The spots are altered cordierite porphyroblasts. Figure 10.2 is a picture of a different biotite hornfels, and Figure 13.24 is a picture of a hornblende hornfels from near Wawa, Ontario. Both formed by contact metamorphism of a pelitic rock.
Rocks of the inner zone of the Onawa aureole were metamorphosed in conditions of the pyroxene-hornfels facies (Figure 14.34). These rocks contain biotite, sillimanite, cordierite, alkali-feldspar, and plagioclase, with occasional andalusite. Muscovite is absent because metamorphic temperature exceeded the muscovite-out reaction. Overall, the minerals in the Onawa aureole are similar to minerals that form during higher-pressure Buchan metamorphism.
14.6.2 Sanidinite Facies

Pelitic rocks metamorphosed under sanidinite-facies conditions are quite rare and are most commonly found as xenoliths within plutons. These pelitic rocks, characterized by the absence of micas, amphiboles, and other hydrous minerals, often contain uncommon or unusual minerals. Typical minerals include sanidine (a high temperature form of K-feldspar), mullite (a rare aluminosilicate), tridymite (a high-temperature polymorph of quartz), and corundum, as well as some more mundane minerals including cordierite and sillimanite. Orthopyroxene and spinel are sometimes present. Because of the high temperature, sanidinite-facies metamorphism of pelites may overlap with partial melting. So, glass is sometimes present. Figure 14.37 is a photo of a sanidinite-facies mullite-cordierite rock from Scotland.
14.7 Migmatites

The rock seen in Figure 14.38 is a migmatite. The term migmatite means mixed rock, which is very apt. Migmatites are common in high-grade metamorphic terranes, and most have an overall pelitic composition. Petrologists have described many different kinds with different textures, but all are rocks that include distinct zones with light-colored and dark-colored lithologies, such as the migmatite in Figure 14.38. We call the light-colored zones leucosomes, and the dark-colored zones melanosomes (leuco means light-colored and melano means dark-colored). Quartz and feldspar make up most leucosomes. Mafic minerals dominate melanosomes.
Pelitic migmatites are of several different kinds and undoubtedly have several different origins. Some probably form by mineral segregation during metamorphism, in much the same way that rocks develop gneissic banding. Still others seem to have formed by intrusion of granitic magmas into darker-colored mafic country rocks. For example, the migmatite in Figure 14.38 contains leucosomes that have textures suggesting they might be deformed veins (intrusions).

Some migmatites seem to have evolved by anatexis (partial melting). If melting occurred, we use the term restite for the dark-colored mafic leftovers. The migmatite in Figure 14.39 is from Namibia. The leucosome below and to the left of the Euro coin includes large crystals of red garnet and dark-colored cordierite that seem to be floating in a mostly quartz and feldspar matrix. These relationships have been interpreted to mean that the garnet and cordierite crystallized from an Al2O3-rich granitic melt that created the leucosomes. The migmatite in Figure 9.7 also has a blobby appearance suggesting it may have formed by anatexis.
Chapter 16 Section 16.3.4 contains many photos of metamorphosed pelitic and related rocks in hand specimen and in thin section. If you want to know what they look like, go there. |
● Figure CreditsUncredited graphics/photos came from the authors and other primary contributors to this book. The petrogenetic grid for pelites and all the AFM diagrams were modified from graphics by Jane Selverstone. The seminal sources are in various publications by Frank Spear and coworkers. Other sources: 14.0 (opening photo) Metapelite outcrop, Marli Miller, geologypics.com |