Western Mesozoic Orogenies
At the end of this chapter, students should be able to:
- Discuss changes to the tectonic setting along the western coast of North America throughout the Mesozoic
- Describe individual similarities and differences between the Nevadan, Sevier, and Laramide orogenies
- Evaluate changes in deformation style and location of deformation in these orogenies over time
Introduction
In the Paleozoic, after the breakup of Rodinia, Laurentia (the name for the continental core of North America) was an island continent near the equator. It was a time of great biological productivity, firstly in the shallow seas left behind by Rodinia’s breakup, and later on land as the first swamps emerged and spread across the continent. Most of the tectonic activity was found in lands that make up the modern-day Eastern Seaboard of the United States, with the Taconian, Acadian, Alleghanian, and Ouachita orogenies helped to build North America and assemble Pangaea. Below is a look at the Earth around the start of the Taconian Orogeny.
Early in the Paleozoic, the western side of North America was a relatively quiet passive margin. The enigmatic Antler orogeny occurred in the Devonian and Carboniferous, but the cause and extent of it are disputed. That was followed by the even more controversial Sonoma orogeny which may have occurred as late as the early Triassic. Evidence for both the Antler and Sonoma orogenies is only found in Nevada. The only other Paleozoic-aged tectonic activity in western North America is the uplift of the Ancestral Rockies, deep-seated uplifts that formed inland, in present-day Colorado and New Mexico. These may be far-reaching byproducts of other orogenies that were going on at the time (Ouachita or Sonoma orogenies).
Nevadan Orogeny

By Actualist via Wikimedia.
The first major orogenic event in the Mesozoic of North America was the Nevadan orogeny. This occurred on the other side of North America from the breakup of Pangaea and started later, in the mid-Jurassic. At some point prior to the Nevadan deformation, an active margin had developed with a volcanic arc along the west coast. These volcanic rocks and related sediments were deformed with the accretion of numerous terranes from the ancient Pacific Ocean, brought eastward as the Farallon Plate (along with the Kula plate) subducted beneath the North American Plate. The Farallon Plate used to exist between the Pacific Plate and the North American Plate.
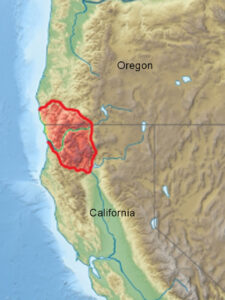
By Alexrk2 via Wikimedia.
The Farrallon’s western boundary with the Pacific Plate was divergent: it was a spreading center (an oceanic ridge). The Farallon’s eastern boundary (with the North American Plate) was convergent: a subduction zone. There, the Farallon was recycled into the mantle. Any island arcs, microcontinents, or oceanic plateaus it was carrying were too thick, buoyant, or protruding too much to subduct. When they reached the subduction zone, these terranes were scraped off and pushed onto the North American continent, adding to its volume. As they were pushed eastward, they acted like a bulldozer, scraping and shoving the volcanic and sedimentary rocks that were layered atop the continental crust. The deformation of the Nevadan orogeny is most easily seen in the Sierra Nevada mountains of California and the Klamath mountains along the coast of California and Oregon.
Marbles of the Boyden Cave Roof Pendant show the results of deformation culminating with batholith emplacement. In addition to the intricate folding displayed here, don’t forget to appreciate the patterns that recent weathering has superimposed on the exposure.
Did I Get It? – Nevadan
Sevier Orogeny
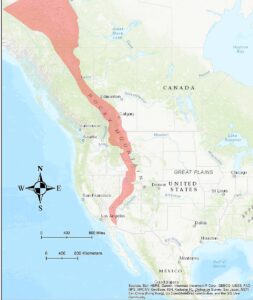
Image by Quickdraw123 via Wikimedia.
The next orogeny to occur in western North America was the Sevier orogeny. While terranes still played a factor in the deformation, the Sevier orogeny occurred much further inland and was more widespread than the Nevadan orogeny. The earliest events associated with the Sevier orogeny are as early as 160 Ma (even before the Nevadan) and the latest deformation was 50 million years ago. However, the majority of this deformation occurred between 120 and 80 million years ago. Subduction at this time changed due to the fact that the Farallon plate was hot and young, causing a Chilean-style subduction zone.
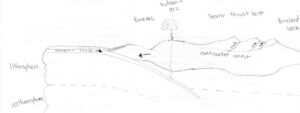
Drawing by Pinkcorundum via Wikimedia.
This means the resistance to subduction caused extra convergent forces in the overriding plate, mainly in the form of a fold-and-thrust belt, which extended inland. Much like the modern Andes, this created a two-ridged mountain chain, with the volcanic arc positioned closer to the trench, and a back-arc mountain range formed from compressional, low-angle thrust faulting. Between these two ridges today is a high plain, called the Altiplano in the Andes. While the exact geography of the mountains during Sevier time is unknown, it can be assumed that it was similar. Igneous rocks, closer to the Sierra Nevada, and shoved stacks of sedimentary rocks far from their original positions deep in the crust and further to the west back up this assertion. These displaced layers of rock arched upward and broke, with the slabs of older rock traveling along thrust faults up on top of younger rocks.
Consider the nascent mountain-building evident in western North America in this paleogeographic depiction from 105 Ma:
Those mountains were driven by compression from the west. As new terranes accreted to the continent, they pushed the pre-existing sedimentary layers out of the way, so that direction was to the east. This process initiated during the Jurassic period of geologic time, but reached its zenith during the Cretaceous. Most of the Farallon Plate was subducted during that time, but two remnants persist into the present day: the Cocos Plate, currently being subducted beneath Central America, and the Juan de Fuca Plate, currently being subducted beneath the Pacific Northwest states of Washington, Oregon, and northern California.
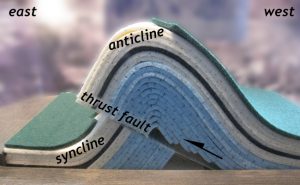
When the sedimentary layers were compressed, they buckled and broke, shearing to the east as they deformed. The result was an immense number of asymmetric folds with the short limb on the east and the long limb on the west, frequently breaking at the hinge of the fold, and initiating a fault. These distinctive structures are the typical structural ingredient in active and ancient compressional mountain belts around the world. This style of deformation is known as thin-skinned deformation. This is referring to these cover rocks, mostly sedimentary, that are involved in the deformation. Crystalline basement rocks are not typically involved in this process. A great example of this occurs in Glacier National Park.
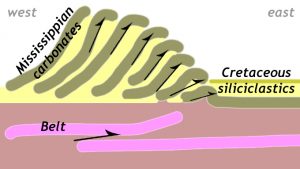
In general with faults, the footwall rocks are those below the fault, while the rocks above the fault are called the hanging wall. However, additional terminology is used when it comes to thrust faults, with their significant lateral offsets, and their orientations so close to horizontal. The block or slab or rock above the thrust fault is sometimes called a thrust sheet, or by the term nappe.
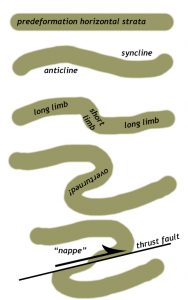
Nappes form when horizontal rock layers are compressed laterally (i.e., from the side) and first buckle into asymmetric folds. The short “limb” of these anticlines and synclines forms in the transport direction (the direction toward which the strata are being thrust). Frequently, this short limb rotates back on itself and becomes tectonically inverted. It also frequently is the place where the strata rupture, and a new thrust fault initiates. Once the mechanical discontinuity of the fault exists, it’s the “weakest link,” and further deformation is accommodated mainly through sliding along that fault surface, not additional folding. Eventually, the fault stops moving, and a new fault forms “outboard” of the first (i.e., further away from the source of the compression). As deformation proceeds, the older thrust sheets get shoved backward and out of the way as new thrusts form beneath them, rising up to the surface. The result is that the older, more western thrust sheets get rotated to steeper dip angles as deformation proceeds. This whole “stack” of nappes is said to be “shingled,” or “imbricated.”
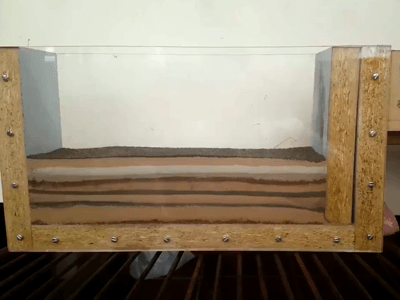
Model and source video by Marco Martins-Ferreira.
Watch the animated GIF here of a sandbox deformation experiment to get a better sense of this process. It’s useful to watch it many times, focusing on a different region each time and watching how it changes with increasing deformation. Imagine that the perspective here is the same as you would see if you were (a) able to go back in time and (b) hover above the crust and look down the axis of the range front, and (c) watch geological processes happen at much faster rates than they really do, and (d) had a magical pair of X-ray glasses that allowed you to peer into the Earth and see these complicated 3D structures evolve in a 2D cross-section.
Laramide Orogeny
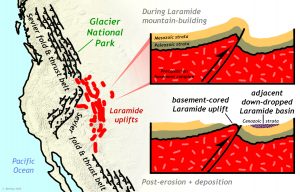
The third mountain-building event that took place in the western US during the late Mesozoic and early Cenozoic, overlapping with but distinct from the Sevier orogeny, is the Laramide orogeny. Ultimately, the Laramide, too, was caused by the subduction of the Farallon Plate. Compared to the Sevier, however, (1) the deformation style was different, (2) the deformation was typically more recent, (3) the deformation took place further to the east, and (4) the deformation was deeper in the crust, involving the crystalline basement. These three variables allow the two orogenies to be distinguished.
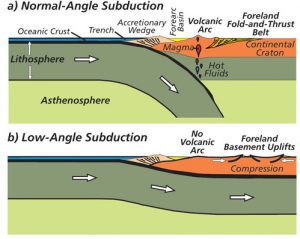
Picture by R. J. Lillie and Parks and Plates by the National Park Service.
The Laramide Orogeny was driven from beneath. Continuing the trend from the Sevier orogeny, the subducting plate was even younger and hotter, causing the subduction angle to become more shallow over time, eventually getting so small that the slab was dragging along the base of the overriding plate. This is known as flat-slab subduction. This subducting but dragging slab is believed to put pressure on the overriding plate, causing warps in the crust to develop. The lack of a mantle wedge below the overriding plate and above the subducting plate also meant that no magmatism occurred during the Laramide orogeny, or at least none related to subduction as it had occurred in the previous orogenies. The onset of Laramide mountain-building was in the late Cretaceous, 80 to 70 Ma, with the activity wrapping up in the window of 55 to 35 Ma. So the two orogenies overlapped in time, but the Sevier started first and ended first.
Deformation style
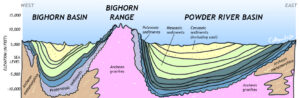
The Laramide orogeny’s style of deformation is thick-skinned, which is a way of noting that the crystalline rocks deep in the crust are very much involved. Laramide deformation is characterized by very large anticlines and synclines that involve basement rock. The anticlines generated highlands and mountain ranges, which were weathered and eroded. The synclines were so significant that they warped the surface of the Earth, generating lowlands. These sedimentary basins that were filled with huge amounts of cast-off sedimentary detritus from the eroding mountains nearby, making new Cenozoic-aged sedimentary deposits that filled the depressions. In some cases, these young sedimentary layers reach thicknesses of 5000 meters! The economically important coal and natural gas deposits of Wyoming formed in Laramide basins, as did the fossil-rich lake deposits of the Green River Formation.
Location
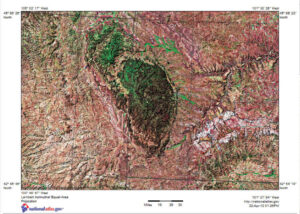
Image by nationalatlas.gov in the public domain.
Laramide deformation does not geographically overlap Sevier deformation. Generally, Laramide deformation is further east. The Black Hills of South Dakota, the Bighorn Mountains of Wyoming, and the Colorado Front Range are all examples of basement-cored Laramide deformation. In contrast, the Sevier fold and thrust belt is further west, in a sinuous arc that runs from the Alberta/British Columbia border through western Montana, Wyoming, Utah, and Nevada, and all the way to Mexico. The Sevier orogen is a much longer feature, too, running twice as long from Canada through the U.S. to Mexico, while the Laramide uplifts are all clustered in the mountain west of the United States alone.
Did I Get It? – Sevier and Laramide
Conclusion
Though the Nevadan, Sevier, and Laramide orogenies are long over, understanding their influence on the geology and topography of the western edge of North America is still crucial today. The Nevadan started as subduction allowed terranes to attach to Laurentia. The Sevier orogeny was an Andean-style subduction zone complete with back-arc thrust sheets. As subduction continued, the bouncy of the subducting plate caused the plate to descend at a shallow angle, causing a much-different Laramide orogeny. This then set the stage for the Basin and Range and the formation of the San Andreas fault.